1-Bit: Ultra Low-Cost, Low-Power Millimeter-Wave Transceiver
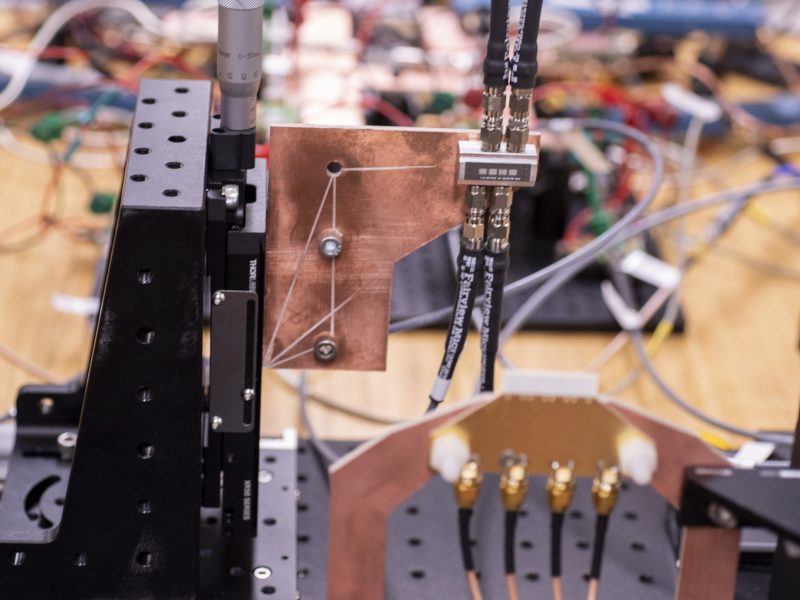
Overview
Fifth-generation cellular network technology (5G) is already here, at least in part. All four major U.S. carriers have rolled out some form of early 5G in the constant race to satisfy public demand for more and better. Consumers want longer battery life, more robust networks, and lower costs for devices and services. They want quicker and more stable access to augmented reality, gaming, and real-time control. In short, they want the next generation of technology before the current one is even mature.
The push for more and better wireless prompted a multi-PI WI team led by Prof. Hochwald to explore the next frontier in wireless communications, specifically millimeter-wave frequencies and gigabit-per-second (Gbps) speeds. Hochwald and his team are collaborating with researchers from AT&T on a 6G project. “The project, titled ‘Ultra-low-cost, low-power millimeter-wave transceivers,’” said Hochwald, “is exploring nontraditional, nonlinear radio architectures in order to create electronics and circuits that can be very low-power and low-cost while not compromising data rates or performance.”
According to Hochwald, the high frequencies of millimeter waves can be both a blessing and a curse. There is an abundance of bandwidth available to support high data rates. However, the technology that operates in these bands is costly and consumes large amounts of power. Additionally, millimeter-wave frequencies have trouble penetrating walls and doors and propagating over long distances. For example, handsets that run these frequencies have a very limited range and, often, short battery life.
The project tests theoretical and practical concepts as team members work to build a new functioning communication system. Rather than employing a small number of very highly capable power-hungry circuits, the team is using a large number of simple low-power circuits to achieve their goals. “A key factor in the project,” said Hochwald, “is to exploit nonlinear circuits that have been traditionally avoided in communications systems.”
Recently, real-world measurements using specially designed prototypes developed by Professor Chisum, whose research efforts include millimeter circuits and antennas, and his students in a WI testbed demonstrated the feasibility of this novel project, obtaining very high data rates at very low power. This work was recently published in the IEEE Transactions on Microwave Theory and Techniques (https://doi.org/10.1109/TMTT.2022.3222424) where the design is reported to consume 0.71-mW of power and achieve Gbps data rates with a BER of 1E-3 at a range of 20cm. By including only 11.5dB of RF gain the prototype sustained Gbps data rates with a BER of 1E-5 at 3.7m. The low power consumption and long ranges achieved with this prototype show it is suitable for scaling to hundreds or thousands of elements in massive multi-in–multi-output (MIMO) arrays for next-generation millimeter-wave wireless communications systems.
The Notre Dame Millimeter-Wave Lab is also being used by the team to assess whether the new transceivers can be competitive with other state-of-the-art transceiver circuits in key performance indicators such as throughput, power, and out-of-band emissions. “We are revisiting many of the basic assumptions of linearity that have traditionally been part of communication system analysis for years. For example, Professor Laneman, who is also part of the team, is helping with the new nonlinear communication theory that is needed to analyze these low-power circuits,” said Hochwald.
Sponsors and Collaborators
Notre Dame team members include Hochwald; Jonathan Chisum, associate professor of electrical engineering; J. Nicholas Laneman, professor and co-director of the WI and Director of SpectrumX.
Electrical engineering graduate students Himanshu Sharma and Xiangbo Meng are currently working on the project. Former graduate students Nick Estes and Kang Gao (Qualcomm) were involved in the project.